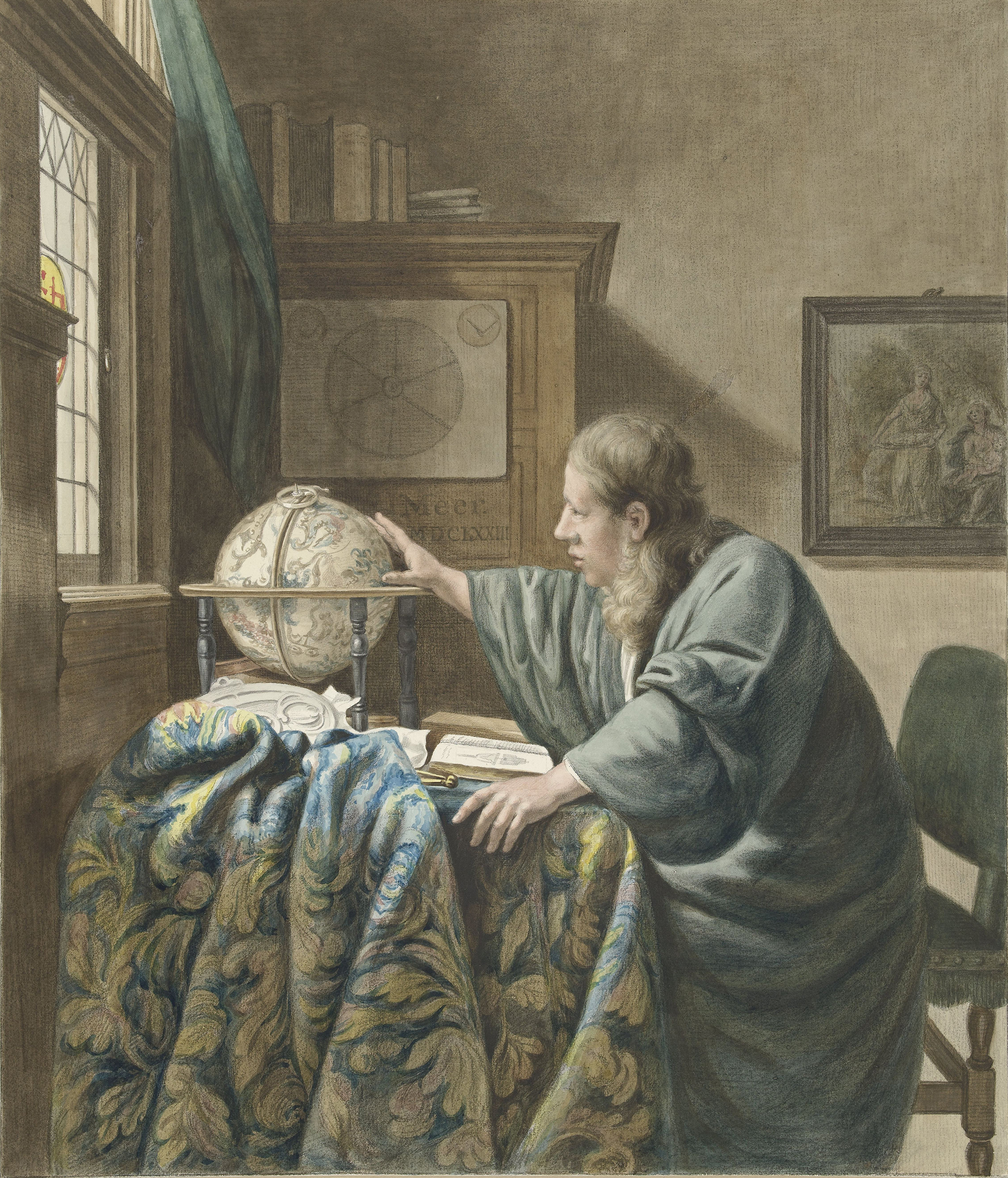
The Astronomer, by Abraham Delfos, 1794. Rijkmuseum.
Alistair Crombie thought medieval science didn’t get enough respect. Around the halfway point of the twentieth century, he wrote a book aimed at correcting the common misperception that the Middle Ages was a dark time for science and investigating nature. Crombie’s own studies had convinced him that much more had been accomplished in the early centuries of the second millennium than other historians (and scientists) had generally recognized.
The so-called dark ages preceding the sudden birth of modern science had in fact, Crombie maintained, been the prelude to the Age of Enlightenment. Of special importance, he believed, was the scientific philosophy and methodology developed at the University of Oxford by the brilliant polymath Robert Grosseteste. “What seems to be the first appearance of a clear understanding of the principles of modern experimental science is found in the writings of the English logician, natural philosopher, and scholar Robert Grosseteste,” Crombie wrote. “In the thirteenth century the Oxford school, with Robert Grosseteste as its founder, marks the beginning of the modern tradition of experimental science.”
Most other historians disputed Crombie’s contention. A more recent assessment by the late David Lindberg considered it seriously extreme. “No informed scholar of my acquaintance accepts Crombie’s claim that Grosseteste was the founder of experimental science,” Lindberg wrote. Crombie himself acknowledged in later writings that he had overstated his case: “Some of the expressions I used about the extent of the medieval contributions to the structure and methods of research of modern experimental science now seem to me exaggerated.”
But Crombie was not wrong to say that the intellectual achievements of early medieval Europe had been neglected and that the scientific enterprise in the Middle Ages was more vigorous and sophisticated than commonly portrayed. And as Lindberg assented, Crombie was also on target in suggesting that Grosseteste was a superstar of medieval science, even if not the founder of modern methods. To those aware of him, Grosseteste is a legendary, larger-than-life character. “Truly Grosseteste was one of the great encyclopedic thinkers of the world,” his biographer Francis Seymour Stevenson wrote in the nineteenth century.
Grosseteste was regarded as an expert on virtually every field of knowledge. He wrote influential treatises on sound and heat, comets, rainbows, and optics. He was also an early cosmologist, proposing a scenario for creating the entire Aristotelian universe—the series of concentric spheres that carried the planets and stars around the earth (then believed to reside at the cosmic center). In proposing a mechanism for constructing the cosmos, Grosseteste also unwittingly outlined a scheme capable of creating a multiverse. Just as late twentieth-century theorists forged an explanation of cosmic origins that implied multiple universes, Grosseteste’s attempt at an origin theory for the thirteenth century’s version of the universe revealed a way for making many other different universes as well.
As with most medieval legends, many aspects of Grosseteste’s life are hard to verify. It seems that he was born in Stowe, County Suffolk, sometime around 1170. His parents were poor, descendants of Normans who came to England with King William’s conquest. Consequently Grosseteste’s native language was French. But he no doubt learned English as well, and his scholarly writings were, of course, in Latin. Apart from evidence for his family’s poverty, no information exists about his youth and early education. He may have attended a cathedral school at Hereford. There are hints that he studied law and also medicine. He certainly studied theology, probably in Paris.
Eventually he made his way (or returned) to Oxford, where he became chancellor—by some accounts, the university’s first. “He was the one really outstanding personality the university produced during the first generation of its existence,” philosopher James McEvoy wrote.
Little else is known of Grosseteste’s early career. And much that some past biographers claimed about it is probably wrong, modern scholars have shown. But whatever the details of his educational background and accomplishments, there’s no doubt that Grosseteste was a man of prodigious intellect and enormous integrity. In 1235 he became the Bishop of Lincoln, making him one of the most prominent and powerful church officials in England until his death in 1253. Much of his reputation over subsequent centuries stemmed from his role in religious issues. He was a voice against corruption and condemned church politics when they conflicted with Christian teachings. He even argued with the pope. Grosseteste’s personal integrity in dealing with affairs of church and state mirrored the intellectual integrity with which he investigated the natural world. His work as a scholar was deeply influenced by the writings of Augustine of Hippo, known to us as Saint Augustine, who in turn had assimilated much of the philosophy of Plato. Grosseteste was also one of the first medieval scholars in western Europe to study Aristotle thoroughly. “Grosseteste…tended to be curious about the historical origins of ideas and the kind of men who had originally thought them up,” McEvoy wrote.
Grosseteste was not blindly devoted to Aristotelian authority, though. Observations settled questions about nature’s behavior, Grosseteste believed, not Aristotle’s writings. Grosseteste also emphasized the importance of mathematics for intellectual rigor in investigating nature. In particular he stressed the key role of geometry (lines, angles, and figures) in the study of optics, which in those days was considered a—or even the—fundamental science, much like particle physics or cosmology today.
He did not possess the mathematical apparatus that is now available for describing the cosmos. But he did attempt his own version of a creation cosmology, built on the notion of light as the fundamental principle of existence. In his treatise on light, he described a process for building a universe—not the universe as we conceive it today, of course, but rather the medieval conception of the universe descended from Aristotle.
Grosseteste sought to explain the origin of a cosmos that had first been envisioned by early Greek philosophers. They perceived need to describe reality rationally, conceiving a “world”—a totality of existence—in which the drama of events proceeded in a regular manner rather than reflecting the chaos of divine whimsy. Those philosophers envisioned “an orderly, predictable world in which things behave according to their natures.” The Greeks called that world the kosmos. Humans have ever since been trying to understand what that kosmos actually is, and how to define it. And whether there is more than one.
Long before the Greek philosophers began their cosmic speculations, skywatchers in Mesopotamia and elsewhere had noticed certain patterns in the movements of the lights dotting the night sky. In their effort to comprehend their kosmos, the Greeks pondered those patterns and tried to infer from them the mechanism of the heavens. Several features of those cosmic motions required explaining: the nightly rotation of the stars around the sky; the appearance of the sun in different segments of the sky during different seasons; the wandering of the planets through the background of the “fixed” stars; and the curiosity of “retrograde motion”—some of the planets appeared to reverse their direction on occasion and move backward.
Before Plato, philosophers had recognized that the stars rotated around the sky as though they were points of light on the inner surface of a sphere. Eventually a consensus emerged that the earth itself was also a sphere, and it seemed that the stars and other heavenly bodies somehow rotated around it. Assuming the earth to be a sphere, Greek astronomers could then define a celestial equator—the plane that passes through the earth’s equator. It was obvious that the sun did not orbit the earth in that plane. Instead it circled at an angle.
The plane of the sun’s orbit—called the ecliptic—was offset from the celestial equator by an angle of 23 degrees. (Today, of course, we know that the earth’s axis is tilted by that angle as it orbits the sun, rather than the sun orbiting the earth at that angle.) For both ancient and medieval astronomers, the relationship between the equator and the ecliptic guided the analysis of heavenly motions.
One particularly peculiar motion complicated the cosmic picture. If you imagine the equator’s polar axis—the line extending upward perpendicular to the equatorial plane and passing through the center of the earth—you’d find that it doesn’t maintain the same position with respect to the axis of the ecliptic. Rather, the polar axis for the equator seems to rotate about the ecliptic axis. Because of that rotation, the points where the ecliptic intersects the equator (marking the spring and autumnal equinoxes) change over time, returning to any given spot every 26,000 years. (The Greeks didn’t have that number exactly right; they thought it was more like 36,000 years.)
In Plato’s time it remained unclear exactly how to account for these motions of the sun, moon, and other planets. A lover of mathematics (and worshipper of the planets as gods), Plato challenged astronomers to ascertain the mechanisms that produced the observed celestial phenomena. As circular motion was divine motion, Plato insisted that the goal be achieved by identifying some combination of uniform circular motions.
Grosseteste approached cosmology not as an astronomer, interested in saving the phenomena, but as a natural philosopher.
While philosophers such as Aristotle had inquired into physical explanations of heavenly motions, Grosseteste took a major step beyond. He wanted to figure out how all that cosmic machinery came into existence in the first place. That was no problem for Aristotle, who thought the cosmos was eternal and everlasting, without beginning or end. Grosseteste, though, operated in the theological framework of Christianity, in which God had created the cosmos—by letting there be light. Grosseteste’s motivation was to reconcile the Aristotelian view with the scriptural importance of light that Augustine had emphasized. Grosseteste believed, correctly, that light emitted at a point emanates outward in all directions spherically. Just as a stone dropped into a pond propagates concentric waves away from the impact, striking a match sends light waves outward in an ever-expanding spherical shell. “Light of its very nature diffuses itself in every direction in such a way that a point of light will produce instantaneously a sphere of light of any size whatsoever, unless some opaque object stands in the way,” Grosseteste wrote in his treatise De Luce (On Light), probably written around 1225. That feature of light suggested to him an idea with at least a superficial similarity to the modern Big Bang theory of the universe’s birth.
Dimensionality—that is, three-dimensional space itself—might have come into existence by the action of light expanding from a point. At the core of Grosseteste’s theory for explaining existence in terms of light is the Aristotelian concept of form. Aristotle believed that pure matter (from which all material things are made) was formless, without meaningful existence. For Aristotle, bodily existence—or corporeity—requires matter plus form. Form imbues formless matter with properties that dictate its nature, and therefore its behavior.
Matter possesses only the potential to become a real body; objects with sensible properties (that is, properties apparent to the senses) are always combinations of form and matter. Grosseteste believed that pure matter did in fact possess a basic form conferring dimensionality or extension, making it a body—that is, giving it corporeity. This forma corporeitatis was conceived by the Persian Islamic philosopher Avicenna (980–1037), who is probably the source of Grosseteste’s idea. Grosseteste, though, was apparently the first to suggest that the source of corporeity—the prime corporeal entity—was light. Both light and matter start out without three-dimensional existence, Grosseteste reasoned, but light is able to generate corporeity by multiplying itself infinitely as it spherically expands. In the beginning, then, light burst the universe into existence and drove matter outward—light’s 3-D expansion somehow took matter along for the ride.
Light, Grosseteste wrote, extended matter “along with itself into a mass the size of the material universe.” As matter spread outward, its density would diminish. But it could not diminish forever, because that would lead to zero density—a vacuum, which nature (everybody then believed) abhorred. At some minimum density, matter’s expansion could continue no longer. At that point matter took the shape of a spherical shell, with a “perfect” ratio of light to matter. Grosseteste called that first shell the firmament. Inside the firmament, matter remained in a fairly ill-formed state, allowing “the possibility of further rarefaction.” Light from the firmament, Grosseteste proposed, shined inward, back toward the center of the universe. In the reverse of the original process, the inward-shining light dragged some of the leftover matter downward to create a second spherical shell, smaller and denser than the first. And then again, light from the second shell, shining inward, would drag matter with it to create another sphere.
Over and over again, this procedure would create new spheres.
Eventually, though, light would fail to produce more spheres. Light from the innermost sphere, containing the moon, apparently did not possess enough corporeal power to create another perfect sphere. It did, though, produce light-giving fire, from which the other elements—air, water, and earth—then formed as well. “This process…continued in this way until the nine heavenly spheres were completely actualized and there was gathered together below the ninth and lowest sphere the dense mass which constitutes the matter of the four elements,” Grosseteste wrote. All this leftover matter, creating the earth as the central sphere, existed in an imperfect state—subject to change, while the nine heavenly spheres were immutable, impervious to corruption.
Grosseteste told a nice story, but from a modern view it lacks quantitative specificity. It wasn’t that he didn’t appreciate math; he was one of the earliest medieval advocates for the benefits of applying math to nature. “All causes of natural effects must be expressed by means of lines, angles and figures, for otherwise it is impossible to grasp their explanation,” he wrote. His belief in light as the source of corporeity led him to consider optics as the most fundamental science, and optics could be understood only through the geometric mathematics of lines and angles.
“Grosseteste’s conception of the nature of physical reality,” Crombie wrote, “led him to the belief that nature could be properly understood only through mathematics.”
Excerpted from The Number of the Heavens: A History of the Multiverse and the Quest to Understand the Cosmos by Tom Siegfried, published by Harvard University Press. Copyright © 2019 by the President and Fellows of Harvard College. Used by permission. All rights reserved.